Ceramics are widely used as structural biomaterials due to their excellent biocompatibility, hardness, corrosion resistance, and bioactivity. These materials play a crucial role in orthopedic, dental, and biomedical applications, particularly where high strength and wear resistance are required. Unlike metals, ceramics are more brittle but excel in non-load-bearing and load-sharing applications where integration with biological tissues is essential.
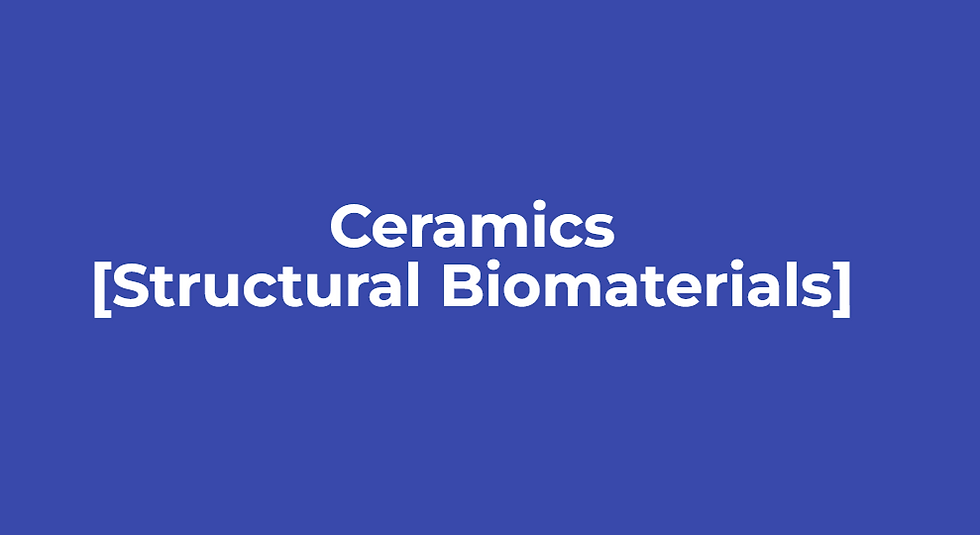
1. Characteristics of Ceramics in Biomaterials
a. Biocompatibility
Ceramics are generally well-tolerated by the body and do not trigger inflammatory or immune responses.
Some ceramics, such as hydroxyapatite, actively support bone growth and integration.
b. Corrosion Resistance
Unlike metals, ceramics do not corrode in bodily fluids, making them highly durable in biological environments.
This makes them ideal for long-term implants in orthopedic and dental applications.
c. High Hardness & Wear Resistance
Ceramics are extremely hard, providing excellent wear resistance, especially in joint replacements.
Their hardness ensures long-term durability, reducing implant wear over time.
d. Brittleness & Low Fracture Toughness
The main limitation of ceramics is their brittleness, meaning they can fracture under excessive stress or impact.
Engineering approaches such as composite reinforcement and microstructural modifications are used to improve their toughness.
e. Bioactivity & Osteointegration
Certain ceramics, like hydroxyapatite (HA) and bioactive glasses, can chemically bond with bone tissue, promoting integration and healing.
This property makes them valuable for bone grafts and coatings for metal implants.
2. Types of Ceramics Used in Structural Biomaterials
a. Bioinert Ceramics (Do not react with biological tissues)
These materials do not bond directly with bone but are used in load-bearing applications due to their strength.
1. Alumina (Al₂O₃)
Advantages:
Extremely hard and wear-resistant.
High corrosion resistance.
Biocompatible and non-reactive with body fluids.
Disadvantages:
Brittle and prone to sudden fracture.
Applications:
Hip joint replacements (as femoral heads).
Dental implants (crowns, abutments).
Bone screws and plates for stabilization.
2. Zirconia (ZrO₂)
Advantages:
Higher fracture toughness than alumina.
Excellent mechanical strength and wear resistance.
Good biocompatibility.
Disadvantages:
Can undergo phase transformation in wet environments, affecting longevity.
Applications:
Dental implants (stronger than alumina).
Orthopedic implants (femoral heads, knee replacements).
Bone screws and spacers.
b. Bioactive Ceramics (Bond directly with bone tissue)
These ceramics promote bone formation and integration, making them useful for coatings and grafts.
3. Hydroxyapatite (HA) [Ca₁₀(PO₄)₆(OH)₂]
Advantages:
Chemically similar to the mineral component of bone.
Supports osteointegration (bone growth and attachment).
Can be used as a coating for metal implants to improve biocompatibility.
Disadvantages:
Brittle and not suitable for load-bearing applications alone.
Applications:
Bone grafts and fillers.
Coatings on titanium implants (hip, dental implants).
Scaffolds for tissue engineering.
4. Bioactive Glass
Advantages:
Bonds with bone by forming a hydroxyapatite-like layer.
Stimulates bone cell proliferation.
Can be used as a bone filler or coating.
Disadvantages:
Low mechanical strength (not used for load-bearing applications).
Applications:
Bone regeneration scaffolds.
Coatings for metal implants.
Dental and cranial implants.
c. Biodegradable Ceramics (Gradually dissolve and get replaced by natural bone)
These ceramics dissolve over time and are replaced by new bone, making them ideal for temporary implants and bone regeneration.
5. Tricalcium Phosphate (TCP)
Advantages:
Biodegradable (resorbs as new bone forms).
Supports bone regeneration.
Disadvantages:
Weaker than hydroxyapatite; used mainly for non-load-bearing applications.
Applications:
Bone graft substitutes.
Tissue engineering scaffolds.
3. Challenges in Ceramic Biomaterials
a. Brittleness & Fracture Risk
Ceramics are prone to cracking under sudden impact.
Solutions:
Zirconia-toughened ceramics (ZTA) to improve toughness.
Ceramic-polymer or ceramic-metal composites to enhance flexibility.
b. Mechanical Mismatch with Bone
Some ceramics are much stiffer than bone, leading to stress shielding.
Solutions:
Porous ceramics that mimic bone structure.
Composite biomaterials that balance strength and flexibility.
c. Slow Degradation Rate (for Bioactive Ceramics)
HA and bioactive glasses integrate with bone but degrade slowly, which may not match natural healing rates.
Solution:
Using biodegradable ceramics like TCP in combination with HA.
4. Applications of Ceramics in Biomedical Engineering
Application | Ceramic Material Used |
Hip & Knee Implants | Alumina, Zirconia |
Dental Implants | Zirconia, Alumina, Hydroxyapatite |
Bone Grafts | Hydroxyapatite, Tricalcium Phosphate, Bioactive Glass |
Coatings for Metal Implants | Hydroxyapatite, Bioactive Glass |
Cranial & Facial Implants | Bioactive Glass, Hydroxyapatite |
Scaffolds for Tissue Engineering | Tricalcium Phosphate, Bioactive Glass |
5. Future Trends in Ceramic Biomaterials
a. 3D-Printed Ceramics
Additive manufacturing allows for the creation of custom implants with porous structures for better bone integration.
Used in patient-specific orthopedic and cranial implants.
b. Composite Ceramics
Combining ceramics with polymers or metals to improve toughness and flexibility.
Examples:
Ceramic-polymer scaffolds for bone regeneration.
Ceramic-metal composites for load-bearing applications.
c. Smart Bioactive Ceramics
Ceramics infused with growth factors, antibiotics, or stem cells to enhance healing.
Bioactive glass composites that stimulate faster bone regeneration.
d. Nanostructured Ceramics
Nano-hydroxyapatite and nano-ZrO₂ provide better bioactivity and mechanical properties.
Improves bone attachment and long-term performance.
Conclusion
Ceramics play a vital role in structural biomaterials due to their biocompatibility, corrosion resistance, and bioactivity. While they excel in wear resistance and bone integration, their brittleness and mechanical limitations remain challenges. Ongoing research in composite materials, 3D printing, and bioactive enhancements aims to overcome these limitations, ensuring ceramics remain a key component in future biomedical advancements.
Next: Polymers
Comments