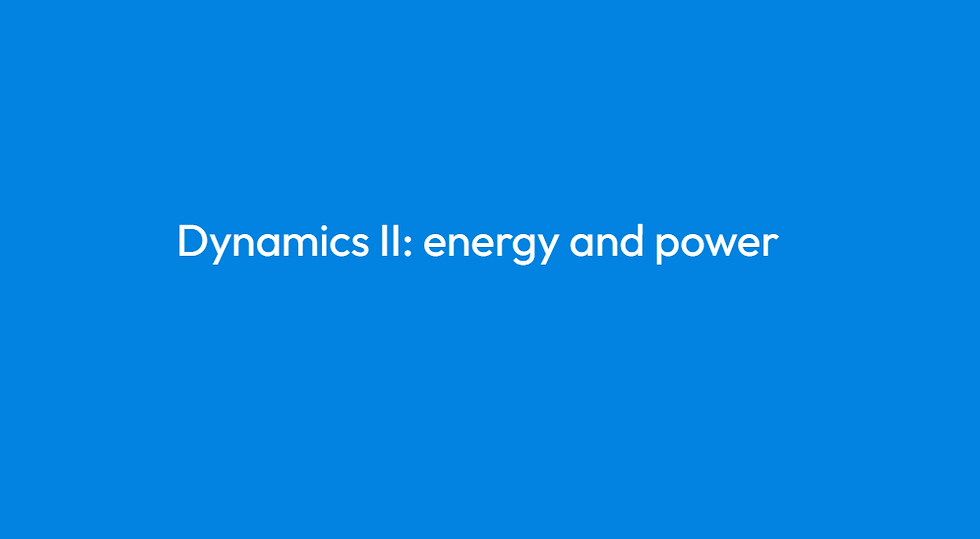
Dynamics II: Energy and Power in Biomechanics
1. Introduction
Energy and power are essential concepts in biomechanics, determining how the body stores, transfers, and utilizes mechanical energy during movement. These principles help in analyzing sports performance, injury prevention, rehabilitation, and prosthetic design.
Energy is the capacity to do work.
Power is the rate at which energy is transferred or work is done.
Understanding energy and power in biomechanics helps in improving movement efficiency, reducing fatigue, and optimizing athletic performance.
2. Mechanical Energy in Human Movement
a. Types of Mechanical Energy
Type | Definition | Example in Human Motion |
Kinetic Energy (KE) | Energy due to motion KE=12mv2KE = \frac{1}{2} mv^2KE=21mv2 | Sprinting, jumping |
Potential Energy (PE) | Stored energy due to position PE=mghPE = mghPE=mgh | Standing at the top of stairs |
Elastic Energy | Energy stored in stretched tissues | Achilles tendon during running |
Kinetic Energy increases with speed and mass (important in sprinting and jumping).
Potential Energy is highest when the body is elevated (e.g., high jump).
Elastic Energy is crucial in sports like running, where tendons store and release energy for efficiency.
3. Work-Energy Principle in Biomechanics
Work is the transfer of energy through force application: Work=Force×DistanceWork = Force \times DistanceWork=Force×Distance
The work-energy principle states that the work done on a body results in a change in its energy.
In biomechanics, work occurs when muscles generate force to accelerate, decelerate, or lift the body.
Example: Work Done in Vertical Jumping
Muscles do work against gravity to store potential energy at takeoff.
Upon landing, energy is absorbed through eccentric muscle contraction.
4. Power in Human Movement
a. Definition of Power
Power is the rate at which work is done: Power=WorkTime=Force×VelocityPower = \frac{Work}{Time} = Force \times VelocityPower=TimeWork=Force×Velocity
Higher power output enables faster movements and explosive actions in sports.
b. Types of Power in Biomechanics
Type | Description | Example |
Positive Power | Energy generation (concentric contraction) | Pushing off the ground in a jump |
Negative Power | Energy absorption (eccentric contraction) | Landing from a jump, decelerating |
Explosive movements (e.g., sprinting, weightlifting) require high power production.
Controlled movements (e.g., landing, braking) involve power absorption.
5. Energy Efficiency & Economy in Human Motion
Energy efficiency refers to how effectively the body uses energy.
Energy economy is crucial in endurance sports (e.g., marathon running).
Stretch-shortening cycle (SSC):
Tendons store and release elastic energy, reducing metabolic cost.
Example: Achilles tendon recoil during running improves efficiency.
6. Applications in Biomechanics
Field | Application |
Sports Performance | Maximizing power output in sprinting, weightlifting |
Injury Prevention | Managing impact forces and energy absorption |
Rehabilitation | Restoring energy efficiency in movement patterns |
Prosthetics & Exoskeletons | Designing energy-efficient assistive devices |
Ergonomics | Reducing energy expenditure in workplace tasks |
7. Conclusion
Energy and power are critical in understanding human movement efficiency, athletic performance, and injury mechanisms. By optimizing energy transfer and power output, biomechanics helps in sports training, rehabilitation, prosthetic development, and ergonomic design, leading to better performance and reduced injury risks.
Comentarios